Living systems are governed by molecular behaviour at the nanometre scale, where chemistry, physics, biology, and computer simulation all now converge.
Implications for Physics:
1. Nanoscale materials mass is extremely small and gravitational forces become negligible. Instead electromagnetic forces are dominant in determining the behaviour of atoms and molecules.
2. Wave-Particle duality of matter: For objects of very small mass, such as the electron, wavelike nature has a more pronounced effect. Similarly, nanoscale materials too exhibit wave behaviour.
3. Quantum confinement: In a nanomaterial, such as a metal, electrons are confined in space rather than free to move in the bulk material. 2D quantum confinement leads to quantum wire and 3D quantum confinement to quantum dot.
4. Quantization of energy: electrons can only exist at discrete energy levels. Quantum dots are nanomaterials that display the effect of quantization of energy.
Fig.
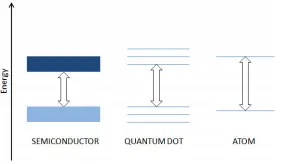
5. Increased surface-to-volume ratio: One of the distinguishing properties of nanomaterials is that they have an increased surface area.
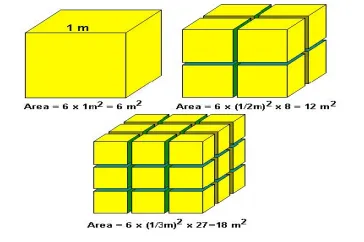
6. Cooling chips or wafers to replace compressors in cars, refrigerators, air conditioners and multiple other devices, utilizing no chemicals or moving parts.
7. Sensors for airborne chemicals or other toxins.
8. Photovoltaics (solar cells), fuel cells and portable power to provide inexpensive, clean energy.
9. Nanoparticle reinforced polymers (or) Nanocomposites:
Requirements for increased fuel economy in motor vehicles, demand the use of new, lightweight materials — typically plastics — that can replace metal. Nanocomposites, a new class of materials, consist of traditional polymers reinforced by nanometre-scale particles dispersed throughout. These reinforced polymers present an economical solution to metal replacement. These nanocomposites can be easily extruded or molded to near-final shape, provide stiffness and strength approaching that of metals, and reduce weight. Corrosion resistance, noise dampening, parts consolidation, and recyclability all would be improved. The weight reduction of motor vehicles from proposed potential applications are expected to save fuel and thereby reduce carbon dioxide emissions. (~15 billion liters of gasoline is expected to be saved over the life of one year’s production of vehicles by the American automotive industry and thereby reduce carbon-dioxide emissions by more than 5 billion kilograms).
10. Nanocomposite materials also find use in non-automotive applications such as pipes and fittings for the building and construction industry; refrigerator liners; business, medical, and consumer equipment housings; recreational vehicles; and appliances.
11. The current generation of lithium ion batteries will be replaced by nanotechnology power sources. Because lithium ion batteries work just fine for a cell phone used for the occasional short phone call. However, if used to power future smart phones, such a battery is likely to run down quite quickly. Nanotechnology will help enable new kinds of power sources, such as better batteries, miniature fuel cells, and tiny photovoltaic panels that will have greater power densities than today’s batteries. It will also enable more energy efficient components and sub-assemblies for mobile devices. For example, a new generation of thin-film transistors built using organic molecules is enabling low-power plastic displays. Displays are typically the most power consuming subsystem in mobile computing or communications equipment. In addition to saving power, nanotechnology has the potential for bringing down the cost of mobile terminals and increasing the quality of visual output from these terminals.
Implication for Chemistry:
1. Nanomaterial is formed of at least a cluster of atoms or cluster of molecules. It follows all types of bindings that are important in chemistry which are important in Nanoscience. They are generally classified as:
Intra-molecular bonding (chemical interactions):
These are bonding that involve changes in the chemical structure of the molecules. They include: ionic bonds, covalent bonds and metallic bonds;
Inter-molecular bonding (physical interaction):
These are bonding that do not involve changes in the chemical structure of the molecules. They include ion-ion and ion-dipole interactions; Van der Waals interactions; hydrogen bonds; hydrophobic interactions; repulsive forces.
Nanomaterials often arise from a number of molecules held together or large molecules that assume specific three-dimensional structures through intermolecular bonding. In these macromolecules intermolecular bonding often plays a crucial role. The bonds such as hydrogen bonding and Van der Waals bonding are though weak, their total energy is quite large due to large number of interactions. Example: In the structure of DNA (nanoscale), the two helixes are held together by numerous hydrogen bonds. This point becomes particularly relevant in Nanoscience, where materials can have very large surface areas and consequently small forces can be applied to very large areas.
Intermolecular bonding often hold together macromolecules (proteins) in specific three-dimensional structures with which precise biological functions are associated.
2. Nanoscale particles exhibit greater equilibrium vapor pressures, chemical potentials and solubility’s relative to bulk materials. This is due to high surface energy of such small particles. Anything that enhances the prospect of atomic/molecular motion also enhances particle growth and aggregation which in turn is restricted by using molecular caps for such nanostructures to terminate and stabilize the nanostructures.
3. Ionization potential increases as the transition-metal-atom cluster drops to Nano scale. This is mainly applied in heterogeneous catalysis. In chemistry, heterogeneous catalysis refers to the form of catalysis where the phase of the catalyst differs from that of the reactants. Phase here refers not only to solid, liquid, vs gas, but also immiscible liquids, e.g. oil and water.
4. Developed by the oil industry, the ordered mesoporous material MCM-41 (known also as “self-assembled monolayers on mesoporous supports,” SAMMS), with pore sizes in the range of 10−100 nanometres, is now widely used for the removal of ultrafine contaminants.
5. Nanofluidic is the study of the behaviour, manipulation, and control of fluids that are confined to structures of nanometre (typically 1-100 nm) characteristic. Motivation to go from micro-to Nano-electronics drives scientist to integrate and miniaturize chemistry and to try to understand and manipulate smaller and smaller amounts of liquids. This is essential when only small amounts of reactants are available. It also helps to better control potentially toxic or explosive reactions. The idea is to integrate a complete laboratory into a silicon waver or a plastic chip. Although this concept has not penetrated our everyday live to the same extent as microelectronics has, the first commercial applications are meanwhile available, e.g., enzymatic analysis and DNA-sequencing. The channels in which the substances are transported in existing devices have typical diameters of 50-100 µm and are still macroscopic. Correspondingly this technique is called microfluidics.
6. A dendrimer is a synthetic, three-dimensional macromolecule. It is built up from a monomer, with new branches added in steps until a tree-like structure is created (dendrimer comes from the Greek dendra, meaning tree). The largest molecules ever made with an atomically defined structure are the dendrimer shown in the figure below. It consists of precisely 5592 benzene rings and has a molecular mass of 546404 g/mol. Dendrimers can not only be made large. They can also be made with specific functions, such as efficient fluorophores or as carriers, e.g. for drugs. The inner part of the dendrimer provides a defined environment, while the groups on the surface regulate the compatibility with the environment.
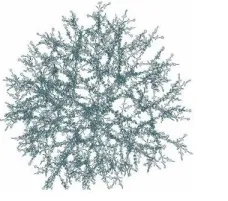
7. Synthesis of nanoparticles : Many materials, which are relevant for novel energy cycles and more efficient chemical reactions (catalysis) do not exist as nanostructures so that “de novo” systems have to be designed from scratch. This for instance holds for metal carbide and nitride particles, which offer new pathways for metal/base catalysis. They also hold the record in mechanical hardness and magnetization. In general, both size and shape add to the favourable properties and must be controlled or adjusted.
8. New cathode nanomaterials for the lithium battery are another target for novel nanostructures where progress will directly impact society
9. Nanoparticles by design: Using emulsion droplets as nanoreactors for precipitation Taylor-made nanoparticles with well-defined size and shape are needed for new applications e.g. in surface physics, catalysis, and biomedicine. Emulsion-assisted precipitation is a very attractive process technology for the production of Taylor-made nanoparticles. In this approach, the droplets of microemulsions (droplet size 2 to 100 nm) or miniemulsions (droplet size > 100 nm and < 1 µm) are used as reaction compartments to perform the precipitation of nanoparticles, initiated by a liquid-phase chemical reaction which is followed by nucleation and growth of solid particles.
10. It is identified that ‘inertness” is not found in few chemical reactions of nanostructure materials due to its high-reactivity character. So, strategies are made to stabilize the nanostructured materials.
11. The construction of nanostructures molecule-by-molecule introduces the distinct advantage that organization and functionality can be manipulated by chemical design. This refers to the spontaneous association of molecules under near-equilibrium conditions into stable, well-defined aggregates joined by non-covalent bonds. It is the key building principle of all living matter and the basics of supramolecular chemistry.
12. Catalysis: A catalyst is a substance that increases a chemical reaction rate without being consumed or chemically altered. Nature’s catalysts are called enzymes and are able to assemble specific and end-products, always finding pathways by which reactions take place with minimum energy consumption. Man-made catalysts are not so energy efficient. They are often made of metal particles fixed on an oxide surface, working on a hot reactant stream. One of the most important properties of a catalyst is its active surface where the reaction takes place. The ‘active surface’ increases when the size of the catalysts is decreased: the smaller the catalyst particles, the greater the ratio of surface-to-volume. The higher is the catalysts’ active surface, the greater is the surface reactivity. Research has shown that the spatial organization of the active sites in a catalyst is also important. Both properties (nanoparticle size and molecular structure/distribution) can be controlled using nanotechnology. Hence, this technology has great potential to expand catalyst design with benefits for the chemical, petroleum, automotive, pharmaceutical and food industries. The use of nanoparticles that have catalytic properties will allow a drastic reduction of the amount of material used, with resulting economic and environmental benefits.
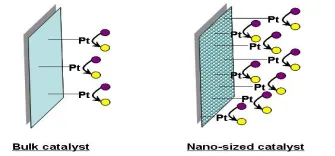
13. Sustainability: Nanotechnology will improve agricultural yields for an increased population, provide more economical water filtration and desalination, and enable renewable energy sources such as highly efficient solar energy conversion; it will reduce the need for scarce material resources and diminish pollution for a cleaner environment. For example, in 10 to 15 years, projections indicate that nanotechnology-based lighting advances have the potential to reduce worldwide consumption of energy by more than 10%.
Implication for Biology:
1. Earlier difficult process of genome sequencing and detecting the genes’ expression can be made dramatically more efficient through use of nanofabricated surfaces and devices.
2 Expanding our ability to characterize an individual’s genetic makeup have revolutionized diagnostics and therapeutics.
3 Nanotechnology can provide new formulations and routes for drug delivery, enormously broadening the drugs’ therapeutic potential.
4 Advanced drug delivery systems, including implantable devices that automatically administering drugs and capable of sensoring drug level.
5 Medical diagnostic tools, such as cancer-tagging mechanisms and “lab-on-a-chip”, real time diagnostics for physicians.
6. Basic studies of cell biology and pathology, to characterize the chemical and mechanical properties of cells (including processes such as cell division and locomotion) and to measure properties of single molecules.
7 Artificial inorganic and organic nanoscale materials are introduced into cells to play roles in diagnostics (e.g., quantum dots in visualization), but also potentially as active components.
8 Nano-engineered gels and other materials are used to replace lost tissue or to provide structure for the regeneration of natural tissue. Current applications include bone replacement and nanostructures that help in the re-growth of nerves.
9 Detection: The detection of a specific chemical or biological compound within a mixture represents the basis for the operation of numerous devices, like chemical sensors, biosensors and microarrays. As with catalysis, a detection reaction occurs at the material interface. The rate, specificity and accuracy of this reaction can be improved using nanomaterials rather then bulk materials in the detection area. The higher surface to volume ratio of nanomaterials increases the surface area available for detection with a positive effect on the rate and on the limit of detection of the reaction. In addition, nanomaterials can be designed to have specific surface properties (chemical or biochemical), tailored at a molecular level. This way, the active sites on the material surface can act as “locks” to detect specific molecules. Scaling down using nanomaterials allows packing more detection sites into the same device, thus allowing the detection of multiple analytes. This scaling-down capability, together with the high specificity of the detection sites obtainable using nanomaterials, will allow the fabrication of super-small “multiplex detection devices”, that is, devices that can test and detect more than one analyte at the time.
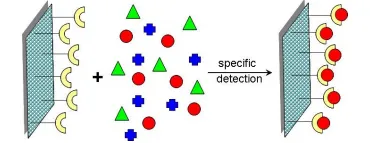
10 The molecular building blocks of life — proteins, nucleic acids, lipids, carbohydrates, and their non-biological mimics — are examples of materials that possess unique properties determined by their size, folding, and patterns at the nanoscale. Biosynthesis and bioprocessing offer fundamentally new ways to manufacture chemicals and pharmaceutical products. Integration of biological building blocks into synthetic materials and devices will allow the combination of biological functions with other desirable materials properties. Imitation of biological systems provides a major area of research in several disciplines. For example, the active area of bio-mimetic chemistry is based on this approach.
11 Nanotechnology will contribute directly to advancements in agriculture in a number of ways: (1) molecularly engineered biodegradable chemicals for nourishing plants and protecting against insects; (2) genetic improvement for animals and plants; (3) delivery of genes and drugs to animals; and (4) Nano-array-based technologies for DNA testing, which, for example, will allow a scientist to know which genes are expressed in a plant when it is exposed to salt or drought stress. The application of nanotechnology in agriculture has only begun to be appreciated.