A reduction in the spatial dimension or confinement of particles or quasi particles in a particular crystallographic direction within a structure generally leads to changes in physical properties of the system in that direction. Hence one classification of nanostructured materials and systems essentially depends on the number of dimensions which lie within the nanometre range.
Nano particles:
· Nanoparticles are particles between 1 and 100 nanometres in size.
· They exhibit three-dimensional confinement. This structure does not permit free particle motion in any dimension.
· Nanoparticles may exist as amorphous or crystalline structure; ie., they may have a random arrangement of the constituent atoms or molecules (amorphous material) or the individual atomic or molecular units may be ordered into a regular, periodic crystalline structure.
· If crystalline, each nanoparticle may be either a single crystal or polycrystalline; ie., it is composed of a number of different crystalline regions or grains of differing crystallographic orientations (i.e., polycrystalline) giving rise to the presence of associated grain boundaries within the nanoparticle.
Properties of nanoparticles:
· A bulk material should have constant physical properties regardless of its size, but at the Nano-scale size-dependent properties are often observed. Thus, the properties of materials change as their size approaches the Nano-scale and as the percentage of atoms at the surface of a material becomes significant.
· An example of the change in physical and chemical properties between gold and gold nanoparticles:
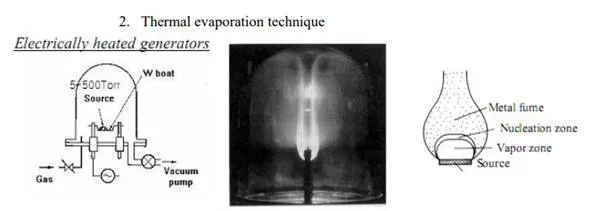
Physical and chemical properties of nanoparticles that may change at the Nano-scale include:
· Colour: Nanoparticles of yellow gold and grey silicon are red in colour.
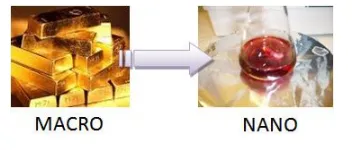
· Melting temperature: Gold nanoparticles melt at much lower temperatures (~300 °C for 2.5 nm size) than the gold slabs (1064 °C).
· Optical Absorption: Absorption of solar radiation is much higher in materials composed of nanoparticles than it is in thin films of continuous sheets of material. In both solar PV and solar thermal applications, controlling the size, shape, and material of the particles, it is possible to control solar absorption. Zinc oxide particles have been found to have superior UV blocking properties compared to its bulk substitute. This is one of the reasons why it is often used in the preparation of sunscreen lotions and is completely photostable.
· Chemical reactivity: Suspensions of nanoparticles are possible since the interaction of the particle surface with the solvent is strong enough to overcome density differences, which otherwise usually result in a material either sinking or floating in a liquid.
· Electrical conductivity: Conductivity of bulk Gold disappears when the particle is reduced to Nano.
· Magnetism: Super-paramagnetism is a form of magnetism, which appears in small ferromagnetic (or) ferrimagnetic nanoparticles. Ferromagnetic materials smaller than 10 nm can switch their magnetisation direction using room temperature thermal energy, thus making them unsuitable for memory storage. (Super Paramagnetic materials are magnetic material with permeability several times greater than that of ferromagnetic materials).
· Mechanical strength: Clay nanoparticles when incorporated into polymer matrices increase reinforcement, leading to stronger plastics, verifiable by a higher glass transition temperature and other mechanical property tests. These nanoparticles are hard and impart their properties to the polymer (plastic).
Synthesis:
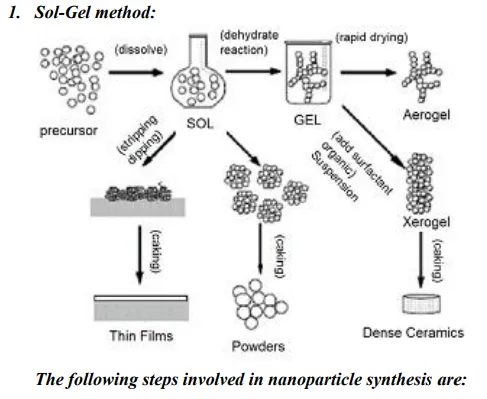
Formation of stable sol solution
· Gelation via a polycondensation or polyesterification reaction
· Gel aging into a solid mass → causes contraction of the gel network, also (i) phase transformations and (ii) Ostwald ripening.
· Drying of the gel to remove liquid phases can lead to fundamental changes in the structure of the gel.
· Dehydration at temperatures as high as 800˚C, used to remove M-OH groups for stabilizing the gel, i.e., to protect it from rehydration.
· Densification and decomposition of the gels at high temperatures (T > 800˚ C), i.e., to collapse the pores in the gel network and to drive out remaining organic contaminants.
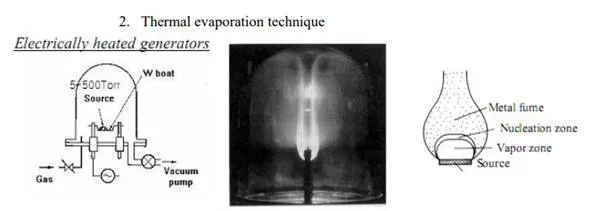
· Electrical heating for evaporation of bulk materials in tungsten heater into low pressure inert gas (He, Ne, Xe)
· Transported by convection and thermophoresis to cool environment
· Subsequent nucleation and growth
· Suitable for substances having a large vapor pressure at intermediate temperatures up to about 1700°C
· Disadvantage: the operating temperature is limited by the choice of crucible
· Evolved to flow process using tubular reactor placed in electrical furnace.
· Requires rapid temperature decrease by the free jet expansion or in a turbulent jet
· Elemental nanoparticles such as Ag, Fe, Ni, Ga, TiO2, SiO2, PbS
Quantum dots:
Quantum dots are extremely small semiconductor structures, usually ranging from 2- 10 nanometres (10-50 atoms) in diameter.
· A quantum dot is a structure that is sufficiently small in all directions that electrons contained on it have no freedom to move in a classical sense and are forced to exhibit quantum characteristics, occupying discrete energy states just as they would in an atom. Indeed, quantum dots have sometimes been referred to as artificial atoms.
· The energy band gap increases with a decrease in size of the quantum dot.
· QDs obey quantum mechanical principle of quantum confinement.
· They exhibit energy band gap that determines required wavelength of radiation absorption and emission spectra.
· Requisite absorption and resultant emission wavelengths dependent on dot size.
· The size, shape and number of electrons can be precisely controlled.
· In some quantum dots even if one electron leaves the structure there is a significant change leaves the structure there is a significant change in the properties.
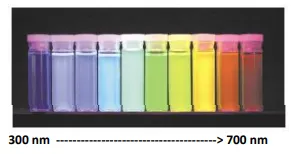
Synthesis: Colloidal method of QD synthesis
Colloidal semiconductor nanocrystals are synthesized from precursor compounds dissolved in solutions, much like traditional chemical processes. The synthesis of colloidal quantum dots is done by using precursors, organic surfactants, and solvents. Heating the solution at high temperature, the precursors decompose forming monomers which then nucleate and generate nanocrystals. The temperature during the synthetic process is a critical factor in determining optimal conditions for the nanocrystal growth. It must be high enough to allow for rearrangement and annealing of atoms during the synthesis process while being low enough to promote crystal growth. The concentration of monomers is another critical factor that has to be stringently controlled during nanocrystal growth. The growth process of nanocrystals can occur in two different regimes, “focusing” and “defocusing”. At high monomer concentrations, the critical size (the size where nanocrystals neither grow nor shrink) is relatively small, resulting in growth of nearly all particles. In this regime, smaller particles grow faster than large ones (since larger crystals need more atoms to grow than small crystals) resulting in “focusing” of the size distribution to yield nearly monodisperse particles. The size focusing is optimal when the monomer concentration is kept such that the average nanocrystal size present is always slightly larger than the critical size. Over time, the monomer concentration diminishes, the critical size becomes larger than the average size present, and the distribution “defocuses”.
There are colloidal methods to produce many different semiconductors. Typical dots are made of binary compounds such as lead sulphide, lead selenide, cadmium selenide, cadmium sulphide, indium arsenide, and indium phosphide. Dots may also be made from ternary compounds such as cadmium selenide sulphide. These quantum dots can contain as few as 100 to 100,000 atoms within the quantum dot volume, with a diameter of 10 to 50 atoms. This corresponds to about 2 to 10 nanometres, and at 10 nm in diameter, nearly 3 million quantum dots could be lined up end to end and fit within the width of a human thumb.
Applications in Medical imaging and diagnostics:
a. QDs can be used as tool for monitoring cancerous cells and providing a means to better understand its evolution.
b. QDs are much efficient than other optical imaging probes such as organic dyes, allowing them to track cell processes for longer periods of time.
c. Quantum dots using its large surface area is well suited for certain kinds of drug delivery.
Quantum dot LEDs:
a. Used to produce inexpensive, industrial quality white light.
b. Produce white light by intermixing red, green and blue emitting dots homogenously than the traditional LEDs.
c. Quantum dot LED’s are extremely energy efficient. They use only a few watts, while a regular incandescent lamp uses 30 or more watts for the same amount of light.
Solar cells and photovoltaics:
A cost-effective third-generation solar cell at better power conversion efficiency is possible by utilizing QDs compared to highly expensive traditional solar cells.